Moore on Moore
We look at the past, present and uncertain future of Moore's Law, with some help from Gordon Moore himself
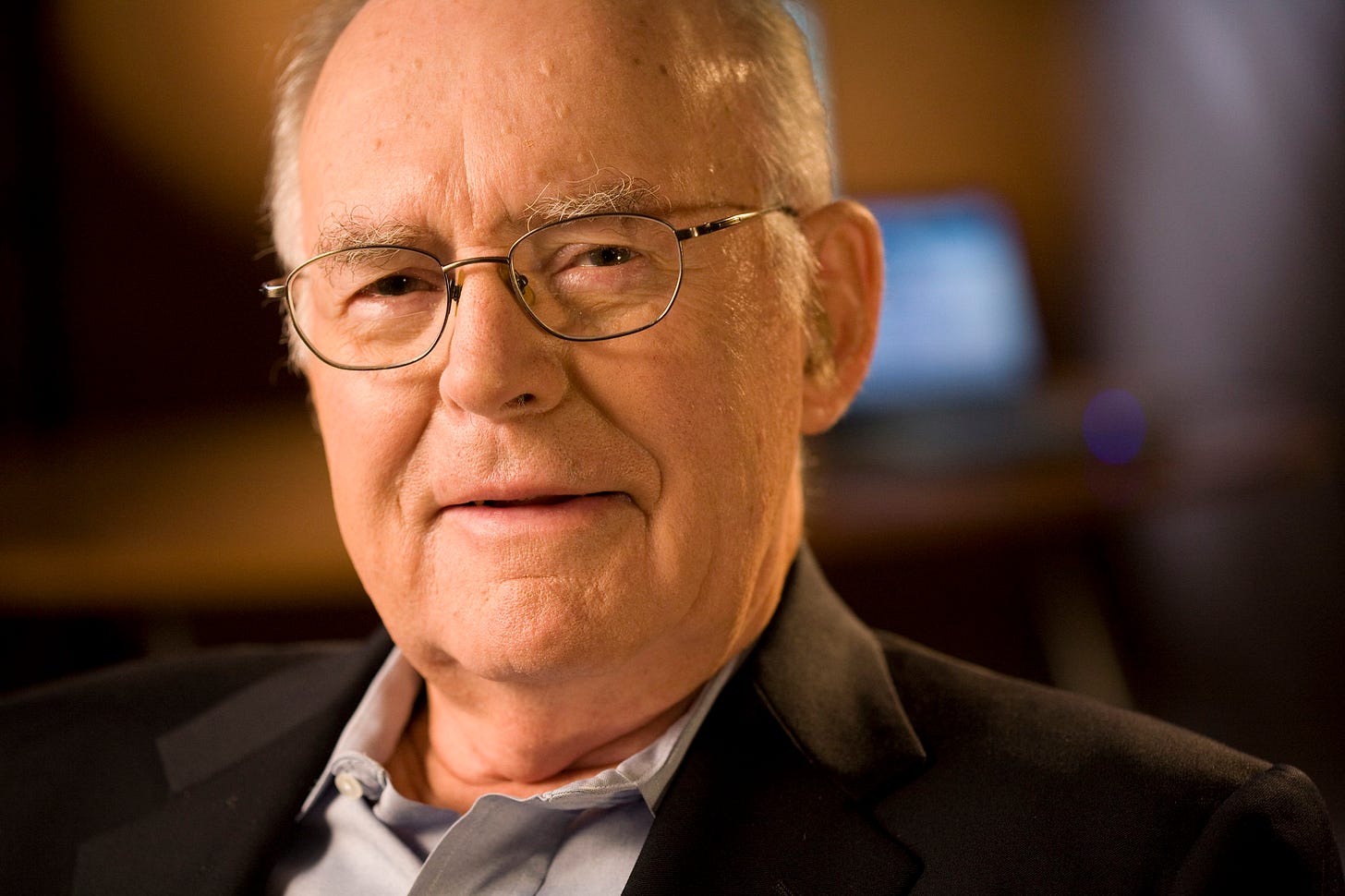
The definition of "Moore's Law" has come to refer to almost anything related to the semiconductor industry that when plotted on semi-log paper approximates a straight line.
Gordon Moore
This post considers the history and current status of Moore’s Law’s. It’s not a detailed exploration of the technical underpinnings of either the history of Moore’s Law or the future development of semiconductors. There are some great sources at the end of the post if you want to go much deeper on this. Rather, it attempts to provide a high-level overview of Moore’s Law and its development. I’ve taken comfort in being able to draw on Gordon Moore’s own views at several points in this exploration. So, with a strong dose of humility …
Two year’s shy of its 60th birthday, Moore’s Law has become a bit like Schrödinger’s hypothetical cat - at once dead and alive.
Economist Newspaper, 16 December 2023
There is a high probability that any discussion of the future of semiconductors will start with Moore’s Law. The recent Economist article ‘Not quite dead yet’1 follows this common pattern.
Moore’s predictions have dominated popular discussion of the topic in recent years. This Google Ngram2 shows how ‘Moore’s Law’ has, over the last decade, appeared in published books almost as often as references to the ‘Integrated Circuits’ that the law describes.
Perhaps we shouldn’t be surprised. As our lives and our societies have been changed by personal computers, the internet, and then smartphones, so ‘Moore’s Law’ has taken on a cultural significance that has reflected the importance of those changes.
But imprecision has crept into many discussions of Moore’s Law. Some commentators have forgotten or choose not to discuss what Moore’s Law really says. The recent Economist article above, for example, presents some important and interesting new technologies, but remarkably, given the name of the newspaper, omits any discussion of the economics that is central to the ‘Law’.
This lack of precision may be at the root of the disagreement alluded to by The Economist. Does Moore’s Law still apply or is it over? Let’s hear from two industry leaders:
“Moore’s Law’s dead” Jensen Huang, Sept 2022
Moore’s Law is “alive and well” Pat Gelsinger, Sept 2022
Jensen says no, and Pat says yes!
So, who is right? We’ll find out more at the end of this post!
This isn’t the first time that Moore’s Law has been proclaimed dead, or at least near the end of its life. Here is a further ngram of the ‘End of Moore’s Law’ which has clearly been a live topic of discussion since the late 1990s.
And here is a quote from Intel’s Mark Bohr:
"The end of Moore's Law is always 10 years away,"
"And yes, it's still 10 years away."
Uncertainty about the future of Moore’s Law is inevitable. If there are foreseeable technical barriers, then doubts about continuation of the Law are to be expected. As we know though, time after time, the semiconductor industry has smashed through those technical barriers.
In this post we’ll look again at Moore’s original and revised predictions. We’ll try to understand what Moore’s Law really means. We’ll look at the various claims about the health of Moore’s predictions today. Finally, we’ll have a brief look at what life might look like after Moore’s Law. And our guide for much of this journey will be Gordon Moore himself.
Before Moore’s Law
Gordon Moore wasn't the first person to predict large increases in the number of transistors on a single integrated circuit. At an IEEE conference in New York in 1964, Harry Knowles from Westinghouse predicted that by 1974:
We’re going to get 250,000 logic gates on a single wafer.
Moore was in the audience and later recalled that he thought Knowles’s prediction was ‘ridiculous’. Fairchild, where he worked, was struggling to fit more than a ‘handful’ of logic gates on a one-inch wafer. Other speakers at the conference also had much more conservative views about the development of semiconductor technology that contradicted Knowles, whose prediction was considered by the others to be ‘wild’.
Moore’s Law (1965 Version)
Yet, Moore was soon to discover that the Knowles prediction wasn't as wild as he’d thought. When he was asked to write an article for ‘Electronics’ magazine in 1965 about the future of semiconductor industry, he looked at his data about what had already been achieved:
I saw that the minimum cost per component point had been coming down quickly over several years, as the manufacturing technology improved. From this observation, I took my few data points and plotted a curve, extrapolating out for the ten years I had been asked to predict.
Moore would later describe his approach in more detail:
Adding points for integrated circuits starting with the early "Micrologic" chips introduced by Fairchild, I had points up to the 50-60 component circuit plotted for 1965 …. On a semi-log plot these points fell close to a straight line that doubled the complexity every year up until 1965. To make my prediction, I just extrapolated this line another decade in time and predicted a thousand-fold increase in the number of components in at the most complex circuits available commercially.
The essence of that extrapolation would form the core of ‘Moore’s Law’:
With unit cost falling as the number of components per circuit rises, by 1975 economics may dictate squeezing as many as 65,000 components on a single silicon chip.
Gordon Moore
Cramming more components onto integrated circuits
Electronics, Volume 38, Number 8, April 19, 1965
Not quite what Westinghouse’s Knowles had predicted but still ‘wild’.
Moore later said that he didn’t expect that this prediction would be followed with much precision:
I was just trying to get across the idea this was a technology that had a future and that it could be expected to contribute quite a bit in the long run.
If we take the numbers that Moore quoted, we have 50-60 components in 1965 increasing to 65,000 in 1975, an increase of around 1,000 in ten years. This amounts to a doubling of the number of components every year over ten years.
Moore’s Law (1975 Version)
One of Moore’s friends, Carver Mead of CalTech, would soon dub this prediction as ‘Moore’s Law’. In 1975, Moore would revisit3 ‘Moore’s Law’. Looking at his latest data he came up with a revised prediction:
The new slope might approximate a doubling every two years, rather than every year, by the end of the decade.
Moore then used this new slope to extrapolate for another ten years, to 1985.
There is also a version of this ‘1975 Law’ that involves doubling (of computer performance) every 18 months, which Moore attributes to Intel’s Dave House:4
Now the one that gets quoted is doubling every 18 months...
I think it was Dave House, who used to work here at Intel, did that, he decided that the complexity was doubling every two years and the transistors were getting faster, that computer performance was going to double every 18 months... but that’s what got on Intel’s Website... and everything else. I never said 18 months that’s the way it often gets quoted.
We’ll stick with Moore’s own prediction for the rest of this post.
Moore’s Law in Practice
So how have Moore’s predictions turned out?
Gordon Moore himself revisited his forecasts in 1995, in an article entitled ‘Lithography and the Future of Moore’s Law’. He plotted a graph that showed that, although his predictions hadn't worked out precisely, the rate of change was being fairly accurately followed.
Moore would revisit his predictions, yet again, on the occasion of the 40th anniversary of his original prediction. He found, yet again, that his revised forecast was panning out well.
For a more recent update, to 2020, we can turn to the “Our World in Data” website, which presents the historic trend of transistor numbers on CPUs:
In 1965, Gordon Moore predicted that this growth would continue for another 10 years, at least. Was he right?
In the chart, we’ve visualized the growth in transistor density – the number of transistors on integrated circuits – from 1970 onwards.
It looks strikingly similar to Moore’s simple plot from 1965. Note again that the transistor count is on a logarithmic axis, so the linear relationship over time means that the growth rate has been constant.
This means that the growth of the transistor count has, in fact, been exponential.
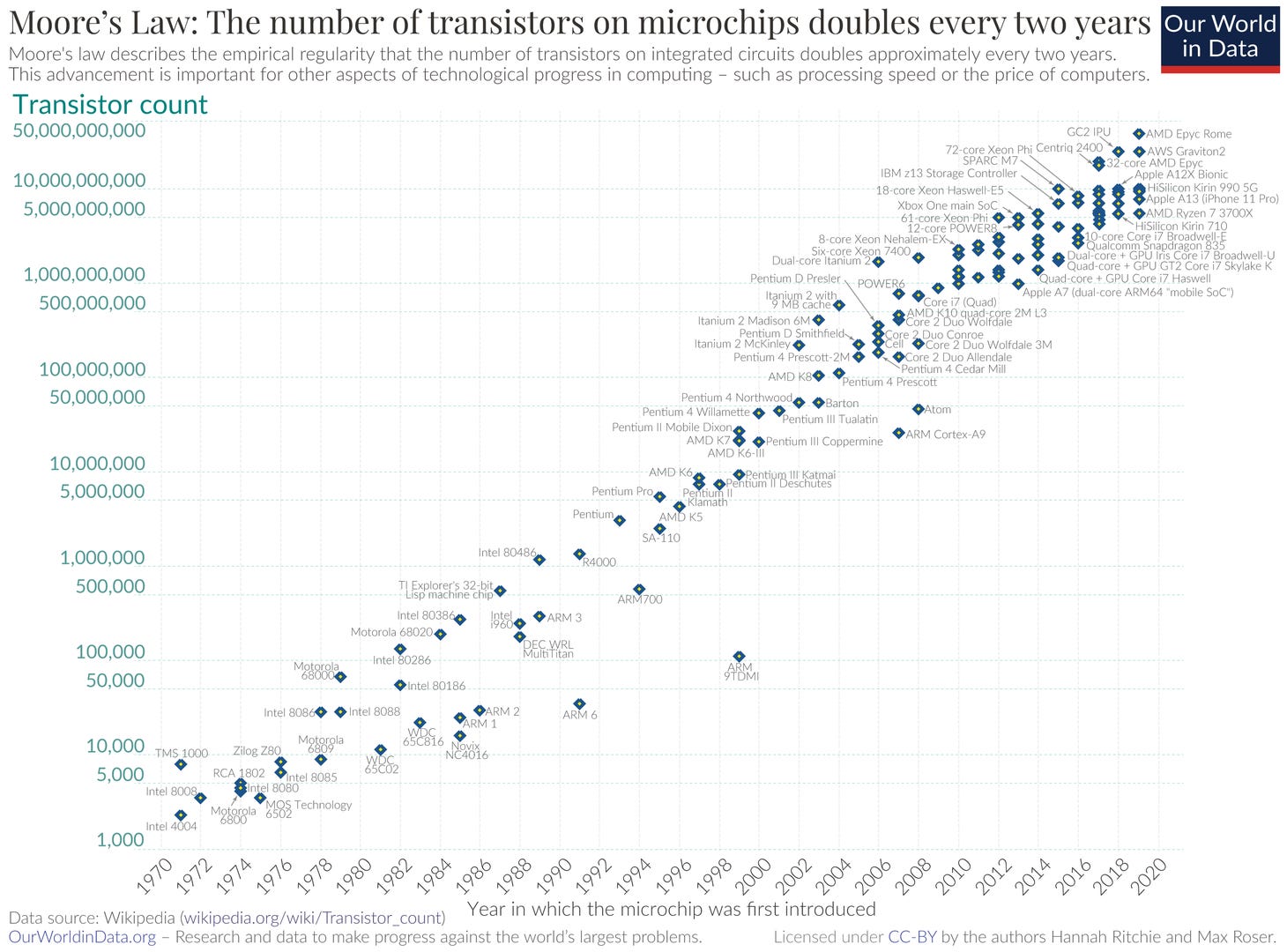
And here is a fun animation which compares CPU and GPU transistor counts, over the period 1969 to 2019, with Moore’s (revised 1975) prediction.
Moore would later comment:
There was no way we could predict very far down the road what was going to happen. It was just a lucky guess, I guess on my part... lucky extrapolation.
So Moore’s predictions have turned out pretty well for a ‘lucky guess’!
How has this progress been achieved?
To get more insight into how Moore’s predictions have worked out in practice it’s worth considering how this progress has been achieved. Moore looked at this in 1975:
He broke improvements down into three components (see the graph above for Moore’s historical analysis and his short term extrapolation from 1975):
Reductions in the size of components;
Increases in semiconductor die sizes;
Contributions from what he called ‘Device and Circuit Cleverness’.
Perhaps the surprise here is how little of the increase was due to the ‘Size Reduction’ contribution, and how much was due to what Moore called ‘Device and Circuit Cleverness’. What was this last item?
This factor I identified with squeezing waste space out of the chip, getting rid of isolation structures and a variety of other things.
He also saw that the contribution from ‘Increases in die sizes’ had been close to the contribution from smaller components. In 1975 an Intel 8080 microprocessor die was 20mm^2. Today, the Apple M1 Max die is 425mm^2. That factor of 20 growth is lower than would be needed to follow Moore’s extrapolation, but still means that it has been a significant contributor to the progression of the Law over the intervening decades.
Moore didn't just look at the growth in die sizes. He also considered increases in wafer sizes, which had grown from three quarters of an inch to 300mm at the time when Moore was writing this retrospective. This didn’t quite match the 57 inch wafer size that one of his conjectured extrapolations would have implied. Intel did provide an illustration of what this might have meant in practice though.
Moore’s Law : Myths and Reality
Before we move on, we should deal with some of the popular myths around Moore’s Law, and emphasise some key points. Moore’s Law:
Is not a ‘natural law’.:
- It’s not really about the underlying physics or chemistry of the devices except that, of course, it’s the underlying physics and chemistry that ultimately constrain how small components can become.Does not predict exponential increases in computer performance.
- We’ve already seen that Moore didn’t predict doubling in performance every 18 months. More components on chips can lead to increases in performance but the relationship is complex and the end of Dennard Scaling5 around 2006 has meant that the rate of increase in performance has slowed even as Moore’s Law has continued.Is not just about shrinking component sizes
- See above and further discussion below.Does make predictions about the progression of the number of components on integrated circuits with the best unit economics i.e the lowest cost per component
- It does not describe the progression of the maximum possible number of components on an integrated circuit.
- We can illustrate this with this chart from Moore’s original 1965 paper which has circuits with more components, but with worse unit economics. Moore’s predictions were about the minima of these curves.
- Crucially, per component costs shrink exponentially. If the cost per component did not fall in this way, then the cost of integrated circuits with exponentially increasing numbers of components would increase exponentially.
As an aside, why does this graph of manufacturing cost per component look like this? There is a clue in a paper from Westinghouse’s Harry Knowles published before the first Moore article. It’s the product of a ‘yield curve’ and ‘100% yield cost per component curve’.
And finally, Moore’s Law:
Has been used to create a timeline around which the semiconductor industry can organise itself.
- Moore’s Law ultimately became a self fulfilling prophesy, in part, as firms organised themselves in such a way as to deliver improvements in line with Moore’s predictions. Perhaps, then, more planning than luck!
Moore’s Law as Economics
If Moore’s Law is not a natural law, then what is it really about? We’ve already had a clue! Let’s go back to Gordon Moore.
Moore’s law is really about economics. My prediction was about the future direction of the semiconductor industry, and I have found that the industry is best understood through some of its underlying economics.
Gordon Moore
Just saying that ‘it’s about economics’ doesn’t really help us to understand what is going on though. One surprise for me was in researching this post was that there seems to have been surprisingly little written about the economics underlying Moore’s Law (any suggestions welcome). Perhaps this is due to the complexity of the topic and the fact that it lies at the intersection of two specialties. Moore’s Law is the end result of a series of highly complex interactions between the economics of semiconductor manufacturing and the underlying technologies.
Trying to cut through this complexity, I think that one (very simplified) way of thinking about Moore’s Law is as the articulation of a virtuous cycle:
Creation of more sophisticated devices … leads to …
A bigger market for the devices … which in turn stimulates …
Investment in R&D and more sophisticated manufacturing … which in turn leads to …
Creation of more sophisticated devices …
… and so the cycle continues.
Moore had seen what pace of technical innovation was feasible within the firms where he worked, at first Fairchild and then Intel. And what was possible depended, in part, on the level of investment that firms could afford to make.
This cycle, as described above, is, of course, a simplification of what happens in practice. It ignores the competition between semiconductor manufacturers which, in practice, will be a major factor influencing their approach to the development of more advanced devices. I think that it’s interesting, though, that according to the model above, competition between firms isn’t a pre-requisite for this virtuous cycle to be sustained.
Another way in which this model is a simplification is that participants can look ahead more than a single two year cycle, anticipate future improvements and prepare for what is needed in later cycles.
And this is one of the wonderful things about the Law. By setting out a timeline for these developments, firms can collectively organise themselves so as to make them happen.
I’m reasonably sure that this was one of the reasons that motivated Moore to set things out in this way. By outlining the pace at which he felt improvements might be expected he gave a prompt to suppliers and customers to prepare for these improvements.
And the actual pace of these improvements is important too. Moore used his observations and his experience to set out a pace of improvement that he felt was likely to be sustainable. If he’d got this wrong then this would be likely to lead to a potential break in the virtuous cycle:
Too rapid a pace would lead to technical over-reach and potential failure to create the more sophisticated devices needed;
Too slow a pace would be inadequate to stimulate the demand which is needed to sustain the investment required to make those devices.
By keeping the pace of progress manageable but meaningful the momentum would be continued.
One incidental benefit of having this pace of improvement made public was that although firms might be tempted to push the pace to gain competitive advantage, the development of the ecosystem at a consistent pace would constrain them.
The End of Moore’s Law
Moore made his last set of predictions in 2005.
It is amazing what a group of dedicated scientists and engineers can do, as the past forty years have shown. I do not see an end in sight, with the caveat that I can only see a decade or so ahead.
Gordon Moore in 2005
We’re almost a decade past that ‘decade or so’ that Moore felt he could see ahead. Can we say more now about when the ‘law’ will come to an end?
The first point to make is that as an exponential like Moore’s Law has to come to an end at some point. There is no way that the number of components on an integrated circuit can continue doubling ‘forever’.
Then, if we go back to our virtuous cycle, we see that this cycle can break due to failure to:
Create more sophisticated devices, or
Create / expand the market for those devices, or
Stimulate investment in R&D and advanced manufacturing
Let’s look at each of these potential ‘points of failure’ in turn.
Physical Limits and Roadmaps
The Economist article that we started this post with focuses on dealing with some of the technological obstacles that lie in the way of creating more sophisticated devices. It highlights a number of measures to try to get around these barriers, ranging from the ‘almost in production’ to the ‘somewhat speculative’, including:
Moving from ‘finFETs’ to ‘Nanosheets’;
Backside power delivery;
Alternatives to Silicon including “transition metal dichalcogenides”;
All of these, one way or another though, are means to one end: shrinking components further.
As we’ve noted Moore’s Law has been used to create a timeline around which the semiconductor industry can organise itself. At this point we can reference the current timeline set out in the ‘International Roadmap for Devices and Systems’ (IRDS).
The executive summary for the 2023 roadmap is freely available for download6 It’s a fascinating, and not too long, read at 64 pages and it provides lots of detail on likely developments in lithography, materials science, metrology and other key aspects of the chip-making process.
We’re not going to try to summarise the contents of the report here. Instead we’ll just look at one aspect of the manufacturing process that might bring Moore’s Law to an end.
Although the ‘headline’ Moore’s Law doesn’t directly specify smaller components, as we’ve seen, in practice, creating smaller components through what are known as ‘node shrinks’ has been key to delivering the exponential increases in components per chip that the law predicts.
At this point we need to clear up one more common misunderstanding. Perhaps the most unhelpful contribution to the public understanding of Moore’s Law has been the naming of ‘process nodes’. In fact ‘node size descriptions’ with labels that are physical lengths, such as 5nm, 3nm, 18A and so on, don’t relate to the actual size of components. It’s not surprising though that there is a common perception that we are coming up to a fundamental limit because of the size of the components are approaching atomic scale. As Samuel K. Moore said in a post subtitled “It’s time to throw out the old Moore’s Law metric” in IEEE Spectrum in 20207:
After all, 1 nm is scarcely the width of five silicon atoms. So you'd be excused for thinking that soon there will be no more Moore's Law, that there will be no further jumps in processing power from semiconductor manufacturing advances, and that solid-state device engineering is a dead-end career path.
You'd be wrong, though. The picture the semiconductor technology node system paints is false. Most of the critical features of a 7-nm transistor are actually considerably larger than 7 nm, and that disconnect between nomenclature and physical reality has been the case for about two decades.
(Samuel) Moore gives an example of what this means in practice:
Gargini, who is chairman of the IEEE International Roadmap for Devices and Systems (IRDS), proposed in April that the industry “return to reality" by adopting a three-number metric that combines contacted gate pitch (G), metal pitch (M), and, crucially for future chips, the number of layers, or tiers, of devices on the chip (T).
“These three parameters are all you need to know to assess transistor density," says Gargini, who also led ITRS.
The IRDS road map shows that the coming 5-nm chips have a contacted gate pitch of 48 nm, a metal pitch of 36nm, and a single tier—making the metric G48M36T1. It doesn't exactly roll off the tongue, but it does convey much more useful information than “5-nm node."
So these components are actually considerably bigger than the node names would seem to imply.
Even so though, these components are still getting very small indeed! Ultimately reach a limit arising from the limitations of EUV lithography.
Of course, we’ve seen limits like this before. EUV was able to break through the previous limit imposed by DUV, but at a cost ….
The Rising Costs of Node Shrinks
That cost takes us to the second potential point of failure, which is the need to create or expand the market for more sophisticated integrated circuits. A caveat first though. What follows is necessarily a hugely simplified discussion of just some aspects of the underlying economics of making chips.
It’s worth noting that, not only have the number of components on integrated circuits increased exponentially, broadly consistent with the law, but these integrated circuits have remained affordable, which in turn implies that per component costs have also shrunk exponentially. That’s in spite of rising costs of semiconductor fabs.
Gordon Moore articulated what has become known as ‘Moore’s Second Law’ or ‘Rock’s Law’ (after Arthur Rock, who helped to fund Intel and was chairman of the company for many years), which states that “the cost of a semiconductor chip fabrication plant doubles every four years”.
Moore himself was acutely aware of the increasing costs of lithography tools. Here is a graph from his 1995 paper:
And, here is a chart of ‘stepper’ prices from US trade body Sematech, drawn at the start of this millennium.
And the increase in the cost of ‘leading edge’ lithography tools has continued to rise rapidly. ASML has just shipped8 its first ‘high-NA’ EUV system to Intel for a reported price of $275m.
These increases in equipment prices over an extended period can be only be sustained if the firms using this equipment are able to grow their sales. And they have. Here is TSMC’s revenue over the last two decades.
Higher Costs and Higher Utility
But what happens if that growth comes to an end. Let’s examine what might happen if higher costs, from more expensive lithography tools, or for other reasons, combined with static demand, ultimately leads to higher prices.
Can higher prices make sense economically? Only if users get corresponding value from these more expensive chips. We can easily identify examples of such sources of value:
Lower power consumption: leading to lower costs over the lifetime of the integrated circuit, or longer battery life for portable devices.
Higher utility: from being able to incorporate more functionality and performance into a single integrated circuit.
At some point, though, the utility of smaller nodes will not be sufficient to justify the higher costs. Even if, consistent with Moore’s Law, these smaller nodes continue to have the best unit economics, they may still imply ‘per chip’ costs that are so high that they cannot justified.
As one example, Apple may, for the moment, be content to pay more for TSMC’s latest wafers, with these chips destined for the most expensive iPhones. But this can’t continue indefinitely though, if prices continue to rise. There is ultimately a limit to what a consumer will pay for a high-end phone.
We then need to remember that rising investment needs increasing demand. Higher wafer costs inevitably reduce that demand breaking the virtuous cycle of greater demand and higher investment that has powered Moore’s Law for decades.
Perhaps there will be a new source of demand for the most advanced semiconductors that will help sustain investment over the long term and keep unit costs down. Maybe from new applications of machine learning? We’ll see.
Ultimately, even if further node shrinks are possible, rising chip costs mean that without that extra demand, the economics of the ‘virtuous cycle’ will break down.
Investment and the ‘Chip Race’
Even if the economics of manufacturing more advanced nodes doesn’t make sense any more, then politics, and particularly geo-politics, may come into play. Here are a few fairly recent headlines:
US reports big interest in $52 billion semiconductor chips funding (Aug 2023)
Brussels approves €8 billion in new subsidies for Made In Europe semiconductors (June 2023)
Japan Prepares $13 Billion to Support Country’s Chip Sector (Nov 2023)
China readying $143 billion package for its chip firms in face of U.S. curbs (Dec 2022)
We’re in a ‘chip race’ at the moment with countries competing to throw money at the creation of new ‘fabs’. And what those countries really want is ‘leading edge’ fabrication technology.
Perhaps, then, the virtuous cycle can be kept in motion for a while by governments funding the investment in R&D and advanced manufacturing needed. I say ‘perhaps’ as there is no certainty that the cash in these headlines, if it is actually spent at all, will be spent well and will advance the state of the art.
And, at some point, even governments will run out of cash, realise that they can’t compete, or fail to see the value in further investment.
Moore himself was acutely aware that exponential growth in demand cannot continue forever. Here is a chart from Moore’s 1995 paper comparing ‘Global Gross World Product’ with the semiconductor industry:
Moore commented on this chart:
As you can see, in 1986 the semiconductor industry represented about 0.1 percent of the GWP. Only ten years from now, by about 2005, if we stay on the same growth trend, we will be 1%; and by about 2025, 10%. We will be everything by the middle of the century. Clearly industry growth has to roll off.
I do not know how much of the GWP we can be, but much over one percent would certainly surprise me. I think that the information industry is clearly going to be the biggest industry in the world over this time period, but the large industries of the past, such as automobiles, did not approach anything like a percent of the GWP. Our industry growth has to moderate relatively soon. We have an inherent conflict here. Costs are rising exponentially and revenues cannot grow at a commensurate rate for long. I think that this is at least as big a problem as the technological challenge of getting to tenth micron.
By my estimate the total revenue of chipmakers in 2023 around a quarter of one percent of global GDP, so Moore was off, in this case, by more than an order of magnitude. His fundamental point still stands though. This relationship must ultimately impose a constraint on how big the industry can grow.
System Integration
Let’s consider one final factor. We’ll return to another observation from Moore’s 1965 paper
As the IEEE article quoted above says:
“Around 2029, we reach the limit of what we can do with lithography," says Gargini. After that, “the way forward is to stack.... That's the only way to increase density that we have."
From the International Roadmap for Devices and Systems executive summary (my emphasis):
Feature scaling will continue unabated for the next 10 years and beyond as a means of increasing transistor density in ICs. However, transistor channel length scaling is no longer a “must do” to meet performance requirement as maximum operating frequency is limited to 5-6 GHz due to limitations imposed by dynamic power dissipation. Multilayer NAND memory cell are solidly in production and nanosheet transistors will follow FinFET transistors and then stacked NMOS/PMOS transistor will follow. A variety of 2.5D and 3D structural approaches will increase components density and integration of many homogeneous and heterogenous technologies in new revolutionary systems.
And building systems out of smaller components doesn’t just mean going ‘vertical’ by ‘stacking’ components. It also encompasses ‘chiplets’ with smaller chips connected side by side.
In the presentation by Philip Wong of TSMC at Hot Chips 2019 entitled “What Will the Next Node Offer Us?”, a presentation that started with this slide:
And then spends more than half of the presentation discussing ‘System Integration’ or creating larger systems out of smaller functions, neatly summarised on this slide:
So once again, almost six decades after his original paper, Moore has proved to be prescient.
Moore’s Law (2023 Version)
Let’s return to the disagreement about the current status of Moore’s Law.
If you’ve been following the debate on Moore’s Law closely then you’ll have spotted that the Pat Gelsinger quote above quite doesn’t represent his latest position on the topic. Just a few months after presenting this slide at Intel Innovate 2023 …
(Note the emphatic “2X every 2 years”.)
… Gelsinger has revised his position to (my emphasis):
Moore's law where essentially you were just able to shrink in the X, Y right and being able to do .7x shrinks in the X and the Y right you're able to get this doubling every two years approximately and that was like the Golden Era of Moore’s law. You know we're no longer in the Golden Era of Moore’s law, it's much much harder now so we're probably doubling effectively you know closer to every 3 years now so we've definitely seen a slowing.
Here is Gelsinger in person (comments above at around 5 minutes):
So today Gelsinger doesn’t really believe that the 1975 version of Moore’s Law is still alive any more (although note that he caveats these comments somewhat later in the talk).
It turns out that the answer to our puzzle as to whether Moore’s Law is alive or dead is that, when we open the box, we will find that Schrödinger’s original unfortunate feline is actually dead. A close relative nearby is still alive though.
In fairness to Gelsinger, a degree of imprecision about what Moore’s Law means has been a feature for a long time. Gordon Moore himself said in 1995:
The definition of "Moore's Law" has come to refer to almost anything related to the semiconductor industry that when plotted on semi-log paper approximates a straight line. I hesitate to review its origins and by doing so restrict its definition.
Let’s try to sum up:
Node shrinks will continue for a while yet at a slower pace but are getting more expensive. But Moore’s Law is not just about shrinking components. There have been and will continue to be other ways to ‘cram more components onto integrated circuits’, including Moore’s ‘device cleverness’ and ‘large systems out of smaller functions’, which will continue to help power (a revised version of) Moore’s Law for a while longer. Finally, it’s quite likely to be economics, and not physics, that ultimately brings Moore’s Law to an end.
After Moore
So what happens then when gains from node shrinks, chiplets, stacking and other sources of improvement really do come to an end? Should we be gloomy? Will technology ‘progress’ come to a grinding halt?
This slide from Nvidia’s Chief Scientist Bill Dally in 2023, via IEEE Spectrum9, tells a different story.
Of a gain of over 1000x in ‘single chip inference performance’ over the last decade only 2.5x of that has come directly from process improvements.
Maintaining the progress of Moore’s Law is the subject of billons and billions of dollars of investment, some very complex engineering, and a bunch of international angst. But it’s only responsible for a fraction of Nvidia’s GPU gains.
In fact ‘number representation’ alone has delivered over 6 times the gains from process improvements10.
This is, of course, related to, what has been called Huang’s Law named after Nvidia’s CEO Jensen Huang:
Huang's law is an observation in computer science and engineering that advancements in graphics processing units (GPUs) are growing at a rate much faster than with traditional central processing units (CPUs)
Perhaps gains like number representation and more complex instructions are ‘one-offs’. They are not part of a pattern of repeated, consistent ‘exponential’ gains such as those delivered by Moore’s Law over more than five decades. Perhaps AI is so new that these big gains are to be expected.
What this misses though is the depth, complexity and the relative ‘newness’ of the whole modern computing stack that is built on top of these fabrication processes. Machine learning may be new, but the rest of the stack isn’t exactly old either.
More than Moore
The Economist, writing in 201611 about the end of Moore’s Law, used an, often-repeated, analogy:
If cars and skyscrapers had improved at such rates since 1971, the fastest car would now be capable of a tenth of the speed of light; the tallest building would reach half way to the Moon.
The Economist suggested that progress would instead defined by progress in three areas : software, with DeepMind’s AlphaGo as an example, the cloud, and by new architectures.
More recently, David Patterson has been talking about ‘A New Golden Age for Computer Architecture’ (see notes for the link to David Patterson and John Hennessy’s Turing lecture):
The end of Dennard scaling and Moore’s Law and the deceleration of performance gains for standard microprocessors are not problems that must be solved but facts that, recognized, offer breathtaking opportunities.
The next decade will see a Cambrian explosion of novel computer archi- tectures, meaning exciting times for computer architects in academia and in industry.
With tens of billions of transistors on a chip there are still many, many opportunities to explore new ways to use them.
Let’s go back to the car analogy. Without their own version of Moore’s Law, cars haven’t got millions of times faster than they were in 1971, but they have improved enormously on a range of other important metrics; safety, comfort, reliability and so on.
And even without shrinking components, the prospects for improvements in computers far exceed those for automobiles. We can look forward to the end of Moore’s Law, in all its forms, with optimism at the prospect of many opportunities that lie ahead.
I’ll leave you with this great (video) teardown of the Cerebras CS212 system, with 850,000 cores - yes, you read that correctly! - just to show what is possible with 2022 technology.
After the break, further reading with lots of links on the past, present and future of Moore’s Law.